Ted Kennedy's Brain Tumor Linked to Cell Phone Use?
Critical thinking? Let's perform a little thought experiment, shall we? If I am right, then avoiding any products involved in these "health scares" will preserve your health and possibly your life. If you are right, then you can continue to consume them with no consequences. But if I am wrong but you act under the assumption I am right, no harm will come to you. However, if I am right but you act under the assumption that I am wrong... it's just your health and life at stake.
When the long term effects of all of these items on human beings and our environment have been exhaustively studied by independent scientists, and have been proven to be safe and any and all evidence of negative impact is conclusively explained away by mitigating factors that have would not be alleviated by avoiding the products in question... then you can conclude they are safe. (Needless to say, the FDA doesn't employ that kind of criteria... otherwise it wouldn't have taken a massive lawsuit to finally get them to admit mercury fillings are dangerous yesterday)
So until that is done, the most logical course of action is to assume they are true and avoid them. For decades the tobacco companies argued that smoking didn't cause cancer, and employed the similar conflicting study arguments. Most of the people who took the common sense, better-safe-than-sorry approach didn't end up with lung cancer, emphysema, black lung, COPD, etc. (unless they breathed in a lot of second hand smoking).
The burden of proof should be that the products are safe. Not that they are unsafe.
There's critical thinking for ya.
_________________
Reasonable people adapt themselves to the world. Unreasonable people attempt to adapt the world to themselves. All progress, therefore, depends on unreasonable people.---George Bernard Shaw
8th Cmdmt: Thou Shalt Not Steal.
But thermal effects are not the only effects of emfs. Going for an X-ray a day would add neglible thermal energy to one's being, yet would significantly increase the risk of various diseases.
Came across another article that said that while the cancer-causing effects are not clearly proven, low energy emf waves can change neural function.
Studying the effects of mobile phone use on the auditory system and the central nervous system: a review of the literature and future directions.
Kaprana AE, Karatzanis AD, Prokopakis EP, Panagiotaki IE, Vardiambasis IO, Adamidis G, Christodoulou P, Velegrakis GA.
Department of Otorhinolaryngology, Head and Neck Surgery, University of Crete School of Medicine, University Hospital of Crete, 71110, Heraklion, Crete, Greece, [email protected].
The wide spread of mobile communication since the late 1980 s raises questions about the effects of electromagnetic fields (EMFs) on the human body. Most studies have focused on the non-thermal effects of electromagnetic radiation (EMR). Low-energy EMFs seem to cause structural and functional changes in the cell membrane of different cell types, leading to abnormal cell response. Such changes within the central nervous system (CNS) and auditory system, which directly receive EMR during mobile phone use, are of particular interest. Various studies suggest that EMR directly affects neurons by reducing the neuronal reactivity, increasing the neural membrane conductivity and prolonging their refractory period. Furthermore, although it has been suggested that EMR is related with increased incidence of specific tumors and can interact with known carcinogenic agents, no conclusive evidence exists supporting its role in carcinogenesis. Therefore, no safe conclusions can be drawn regarding the potential harmful effects of mobile phone use. Experiments are underway by our laboratory to investigate possible effects of mobile phone use on the auditory system and the CNS. These along with other studies are expected to further clarify whether mobile phone use truly presents a health hazard.
Non-thermal activation of the hsp27/p38MAPK stress pathway by mobile phone radiation in human endothelial cells: molecular mechanism for cancer- and blood-brain barrier-related effects.
Leszczynski D, Joenväärä S, Reivinen J, Kuokka R.
Bio-NIR Research Group, Radiobiology Laboratory, Department of Research and Environmental Surveillance, STUK - Radiation and Nuclear Safety Authority, Laippatie 4, FIN-00880, Helsinki, Finland. [email protected]
We have examined whether non-thermal exposures of cultures of the human endothelial cell line EA.hy926 to 900 MHz GSM mobile phone microwave radiation could activate stress response. Results obtained demonstrate that 1-hour non-thermal exposure of EA.hy926 cells changes the phosphorylation status of numerous, yet largely unidentified, proteins. One of the affected proteins was identified as heat shock protein-27 (hsp27). Mobile phone exposure caused a transient increase in phosphorylation of hsp27, an effect which was prevented by SB203580, a specific inhibitor of p38 mitogen-activated protein kinase (p38MAPK). Also, mobile phone exposure caused transient changes in the protein expression levels of hsp27 and p38MAPK. All these changes were non-thermal effects because, as determined using temperature probes, irradiation did not alter the temperature of cell cultures, which remained throughout the irradiation period at 37 +/- 0.3 degrees C. Changes in the overall pattern of protein phosphorylation suggest that mobile phone radiation activates a variety of cellular signal transduction pathways, among them the hsp27/p38MAPK stress response pathway. Based on the known functions of hsp27, we put forward the hypothesis that mobile phone radiation-induced activation of hsp27 may (i) facilitate the development of brain cancer by inhibiting the cytochrome c/caspase-3 apoptotic pathway and (ii) cause an increase in blood-brain barrier permeability through stabilization of endothelial cell stress fibers. We postulate that these events, when occurring repeatedly over a long period of time, might become a health hazard because of the possible accumulation of brain tissue damage. Furthermore, our hypothesis suggests that other brain damaging factors may co-participate in mobile phone radiation-induced effects.
Lepidoptera
Pileated woodpecker
Joined: 9 May 2008
Age: 70
Gender: Male
Posts: 191
Location: Northern California
I think I'm done. I knew this would degrade into a back and forth never ending parade of studies on both sides of the issue. My point in posting was not to convince any of you, but to air another side to the many people who will read this that hear nothing but the alarmist, doom and gloom, we're killing ourselves, corporations are out to get us point of view that the media in it's various forms bombards us with constantly.... and on internet forums too.
I can't imagine living my life with such an outlook. That wouldn't work for me. How's it working for you? Good luck!
LeKiwi
Veteran
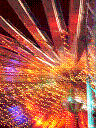
Joined: 26 Nov 2007
Age: 38
Gender: Female
Posts: 2,444
Location: The murky waters of my mind...
I do agree you need to be careful not to get sucked into what I call the 'fear factor' and get terrified over every little thing, because it isn't healthy and what's being used to control people - keep them scared and they'll do what you say. Think about it. How many scare stories do we hear a day? Don't use that, it'll make you sick. Don't use vitamins, they'll kill you. Don't go near arabs, they're all terrorists. Be careful on trains, they'll get blown up. Don't do this, that or the other...
The problem is, most of it plays up to stereotypes and has no grounding in science. People are too scared when they hear something genuinely bad - for example, food additives or fluoride in water or in this case, mobile phone radiation - that they won't change. They're too scared of going against the norm and making that step in the right direction, away from the fear!
It's a very strange situation psychologically and it's very damaging. But surely learning to discern what's right and what's not, and making steps to minimise the things that are genuine hazards, is the best thing to do? I mean, take the vitamins example - they're the very things necessary for life, so why believe the BS 'they kill you' stories? Or in the case of Arabs/Middle Eastern people - it's ludicrous to say they're all terrorists, but that's the assumption we're being brainwashed into, and it's absolutely despicable. These things we're told to fear tend to be things that go against common sense.
On the other hand, you have things people are told constantly are 'safe' that are actually incredibly dangerous - for example, aspartame in food, fluoridated water, aluminium in cosmetics, deodorants and vaccines - and these are the ones that go against common sense, yet people still believe they're safe and are too scared to go against the 'fear factor' and change brands or not buy them.
Common sense has been replaced by common goals. Come on people, open your eyes and start thinking for yourselves again!!
_________________
We are a fever, we are a fever, we ain't born typical...
Yeah, it's called science.
I can't imagine living my life with such an outlook. That wouldn't work for me. How's it working for you? Good luck!
Working fine for me, although I don't consider myself a gloom and doom person. We can set up the threshold so that we only accept things that are very nearly certain, and risk being slow to accept something that is true, or we can accept something that is less certain, and risk having to change our mind when it shown to not be true. Neither strategy will guarantee a better life compared to the other; only in the long run do we win. Peace.
The critical thinking is in evaluating each issue individually rather than either being a paranoid conspiracy freak or being completely oblivious to all potential problems. No need to create a false dichotomy here. I personally don't buy into many of these health scares, as they tend to be over-hyped and I'd rather not get paranoid over what turns out to be nothing.
_________________
WAR IS PEACE
FREEDOM IS SLAVERY
IGNORANCE IS STRENGTH
So until that is done, the most logical course of action is to assume they are true and avoid them. For decades the tobacco companies argued that smoking didn't cause cancer, and employed the similar conflicting study arguments. Most of the people who took the common sense, better-safe-than-sorry approach didn't end up with lung cancer, emphysema, black lung, COPD, etc. (unless they breathed in a lot of second hand smoking).
The burden of proof should be that the products are safe. Not that they are unsafe.
There's critical thinking for ya.
Your argument leads us back into an Amish lifestyle. It frankly could exclude us from ever doing anything because it might carry some risks with it. Hell, we're all sitting here in front of computer screens, how do we know that the little bit of extra background radiation coming from them isn't going to increase our risk of cancer?

_________________
WAR IS PEACE
FREEDOM IS SLAVERY
IGNORANCE IS STRENGTH
So until that is done, the most logical course of action is to assume they are true and avoid them. For decades the tobacco companies argued that smoking didn't cause cancer, and employed the similar conflicting study arguments. Most of the people who took the common sense, better-safe-than-sorry approach didn't end up with lung cancer, emphysema, black lung, COPD, etc. (unless they breathed in a lot of second hand smoking).
The burden of proof should be that the products are safe. Not that they are unsafe.
There's critical thinking for ya.
Your argument leads us back into an Amish lifestyle. It frankly could exclude us from ever doing anything because it might carry some risks with it. Hell, we're all sitting here in front of computer screens, how do we know that the little bit of extra background radiation coming from them isn't going to increase our risk of cancer?

Believe it or not I'm not that paranoid. I smoke a pack a day (proven death trap). I have amalgam fillings in my teeth - dentist put them in before I realized that was what he was putting in... couldn't afford to have him take em out and replace em. I still eat microwave dinners loaded with preservatives. I use my cell phone often. I drink sodas loaded with aluminum and fluoride. However, I am aware of what they do. The dangers of each health risk I mentioned are heavily documented by large numbers of people. Of course those industries fight back with their own studies, and I have already shown how easily the FDA is bought off. Nonetheless, I choose not to live an amish lifestyle, tempting as it may be sometimes. I'm addicted to nicotine, though I am trying to quit. With everything else, I am fully aware that they are killing me... it's just that I don't have a healthy alternative to choose at the moment.
My argument is that we can have our cake and eat it too. There is no reason we can't have vaccines that are free of mercury, formaldehyde and live viruses that cause diseases unrelated to the one they are supposed to cure. There is no reason we have to keep allowing industry to dump their aluminum fluoride waste into our water and charge our cities for it (instead of paying to have it disposed of safely). There is no reason we can't all be driving electric cars charged up by solar panels on the roofs of our houses. There is no reason our milk needs to be full of cancer-causing bovine growth hormone, or that our food needs to be genetically modified without our knowledge. There is no reason we can't have better shielded cell phones. There is no reason any company should ever be allowed to put its profit margin above our lives or health.
There is only one person that can do something about it. He/she is difficult to find though because he/she doesn't keep a high profile. He/she doesn't make a lot of noise yet, but these companies are aware he/she exists. They just don't care what he/she thinks because he/she hasn't made his/her voice heard yet. I know where this leader is hiding though, and I will let you in on the secret of how to find him/her....
Go look into a mirror, and he/she will be the face staring back at you.
Consumer advocates against mercury amalgam fillings won a major victory yesterday. There is no reason we can't follow their lead.
_________________
Reasonable people adapt themselves to the world. Unreasonable people attempt to adapt the world to themselves. All progress, therefore, depends on unreasonable people.---George Bernard Shaw
8th Cmdmt: Thou Shalt Not Steal.
The theory that cell phone use causes cancer is based on junk science.
Anyone can say that about any theory. Creationists say that about evolution. Geocentrists used to say that about heliocentrism.
Can you give us a valid justification to back up your opinion?
_________________
Reasonable people adapt themselves to the world. Unreasonable people attempt to adapt the world to themselves. All progress, therefore, depends on unreasonable people.---George Bernard Shaw
8th Cmdmt: Thou Shalt Not Steal.
Hey! You know what the definition of Junk Science/Pseudo Science is?
It is any kind of science which threatens a corporation's profits. Actually, the real junk/pseudo science is the crap paid for by corporations and performed by "scientists" who are really prostituting themselves for big money and where the outcome has been decided before they even begin the experiment.
A cigarette company executive once said "Doubt is Our Product" and that is exactly what the corporate junk science is meant to do: sow the seeds of doubt and create confusion among the public.
Dr. Henry Lai and Dr. N.P. Singh at the University of Washington proved way back in 1996 that short-term exposure to cell phone radiation broke both single- and double-strand DNA in rat brains. Immediately, the cell phone industry hired Dr. Jerry Phillips, who was working for Motorola and the Department of Energy at the time, to try to prove Dr. Henry Lai and Dr. Singh's findings wrong. Unfortunately for the cell phone industry, Dr. Jerry Phillips got pretty much the same results as Dr. Henry Lai -- i.e. he also found that cell phone radiation broke DNA. And naturally the people at Motorola did not want Dr. Jerry Philips to go public and publish his research findings. He did anyway and naturally no longer works for Motorola.
Phone calls were apparently made to the NIH (National Institute of Health) stating that Dr. Henry Lai was misusing his research funds. They investigated but told him to continue with his research. Letters were apparently also written to the president of the University of Washington demanding that Dr. Henry Lai be fired.
This is the crap that people who are honest and tell the truth have to go through from a trillion dollar industry connected to the US military (who are the ones who originally invented the cell phone by the way). So it is no wonder so many doctors and researchers are afraid to speak out on this issue.
And then there are the dishonest researchers (e.g. Dr. Repacholi, previously with the WHO but now working for the cell phone industry) who prostitute the science and who are more than willing to accept large amounts of money and produce whatever results the cell phone industry wants of them.
And then what do we hear from the media: "The data shows that it is inconclusive." Duh! The only reason they reach this conclusion is due to the fact that there are scientists out there prostituting themselves to the cell phone/military industrial complex and confusing the issue with their pseudo science. And they are supposed to be teaching "critical thinking" skills in our schools. Unfortunately, what they are really only teaching is the ability to regurgitate information with empty cliches like "junk science" or "pseudo science" or "the data is inconclusive."
Copyright © 2009 Elsevier Ireland Ltd All rights reserved.
Electromagnetic fields and DNA damage
J.L. Phillipsa, , , N.P. Singhb and H. Laib
aDepartment of Chemistry, University of Colorado at Colorado Springs, Colorado Springs, CO 80918, USA
bDepartment of Bioengineering, University of Washington, Seattle, WA 98195, USA
Received 24 October 2008; revised 16 November 2008; accepted 16 November 2008. Available online 4 March 2009.
Abstract
A major concern of the adverse effects of exposure to non-ionizing electromagnetic field (EMF) is cancer induction. Since the majority of cancers are initiated by damage to a cell's genome, studies have been carried out to investigate the effects of electromagnetic fields on DNA and chromosomal structure. Additionally, DNA damage can lead to changes in cellular functions and cell death. Single cell gel electrophoresis, also known as the ‘comet assay’, has been widely used in EMF research to determine DNA damage, reflected as single-strand breaks, double-strand breaks, and crosslinks. Studies have also been carried out to investigate chromosomal conformational changes and micronucleus formation in cells after exposure to EMF. This review describes the comet assay and its utility to qualitatively and quantitatively assess DNA damage, reviews studies that have investigated DNA strand breaks and other changes in DNA structure, and then discusses important lessons learned from our work in this area.
Keywords: Electromagnetic field; DNA damage; Comet assay; Radiofrequency radiation; Cellular telephone
Article Outline
1. The comet assay for measurement of DNA strand breaks
2. Radiofrequency radiation (RFR) and DNA damage
3. Extremely low frequency electromagnetic fields (ELF EMF) and DNA damage
4. Some considerations on the effects of EMF on DNA
5. Lessons learned
6. Summary
Acknowledgements
References
1. The comet assay for measurement of DNA strand breaks
DNA is continuously damaged by endogenous and exogenous factors and then repaired by DNA repair enzymes. Any imbalance in damage and repair and mistakes in repair result in accumulation of DNA damage. Eventually, this will lead to cell death, aging, or cancer. There are several types of DNA lesions. The common ones that can be detected easily are DNA strand breaks and DNA crosslinks. Strand breaks in DNA are produced by endogenous factors, such as free radicals generated by mitochondrial respiration and metabolism, and by exogenous agents, including UV, ionizing and non-ionizing radiation, and chemicals.
There are two types of DNA strand breaks: single- and double-strand breaks. DNA single-strand breaks include frank breaks and alkali labile sites, such as base modification, deamination, depurination, and alkylation. These are the most commonly assessed lesions of DNA. DNA double-strand breaks are very critical for cells and usually they are lethal. DNA strand breaks have been correlated with cell death [1], [2], [3], [4] and [5], aging [6], [7] and [8] and cancer [9], [10], [11], [12] and [13].
Several techniques have been developed to analyze single- and double-strand breaks. Most commonly used is microgel electrophoresis, also called the ‘comet assay’ or ‘single cell gel electrophoresis’. This technique involves mixing cells with agarose, making microgels on a microscope slide, lysing cells in the microgels with salts and detergents, removing proteins from DNA by using proteinase K, unwinding/equilibrating and electrophoresing DNA (under highly alkaline condition for assessment of single-strand breaks or under neutral condition for assessment of DNA double-strand breaks), fixing the DNA, visualizing the DNA with a fluorescent dye, and then analyzing migration patterns of DNA from individual cells with an image analysis system.
The comet assay is a very sensitive method of detecting single- and double-strand breaks if specific criteria are met. Critical criteria include the following. Cells from tissue culture or laboratory animals should be handled with care to minimize DNA damage, for instance, by avoiding light and high temperature. When working with animals exposed to EMF in vivo, it is better to anesthetize the animals with CO2 before harvesting tissues for assay. Antioxidants such as albumin and sucrose, or spin-trap molecules such as α-phenyl-tert-butyl nitrone (PBN), should be added during dispersion of tissues into single cells. Cells should be lysed at 0–4 °C to minimize DNA damage by endonucleases. Additionally, antioxidants such as tris and glutathione, and chelators such as EDTA, should be used in the lysing solution. High concentrations of dimethylsulfoxide (DMSO) should be avoided due to its chromatin condensing effect. Treatment with proteinase K (PK; lyophilized DNAse-free proteinase-K from Amresco is ideal) at a concentration of 0.5–1 mg/ml (depending upon cell type and number of cells in the microgel) should be used for 1–2 h at 37 °C to reveal all possible strand breaks which otherwise may go undetected due to DNA–protein crosslinks. Longer times in PK will lead to loss of smaller pieces of DNA by diffusion. Glass slides should be chosen based on which high resolution agarose (3:1 high resolution agarose from Amresco is ideal) will stick well to the slide and on the ability of the specimen to be visualized without excessive fluorescence background. Choice of an electrophoresis unit is important to minimize slide-to-slide variation in DNA migration pattern. A unit with uniform electric field and buffer recirculation should be used. Electrophoresis buffers should have antioxidants and chelators such as DMSO and EDTA. DNA diffusion should be minimized during the neutralization step by rapidly precipitating the DNA. Staining should employ a sensitive fluorescent dye, such as the intercalating fluorescent labeling dye YOYO-1. A cell-selection criteria for analysis should be set before the experiment, such as not analyzing cells with too much damage, although, the number of such cells should be recorded.
There are different versions of the comet assay that have been modified to meet the needs of specific applications and to improve sensitivity. Using the most basic form of the assay, one should be able to detect DNA strand breaks in human lymphocytes that were induced by 5 rad of gamma-ray [14] and [15].
2. Radiofrequency radiation (RFR) and DNA damage
In a series of publications, Lai and Singh [16], [17], [18] and [19] reported increases in single- and double-strand DNA breaks, as measured by the comet assay, in brain cells of rats exposed for 2 h to a 2450-MHz RFR at whole body specific absorption rate (SAR) between 0.6 and 1.2 W/kg. The effects were blocked by antioxidants, which suggested involvement of free radicals. At the same time, Sarkar et al. [20] exposed mice to 2450-MHz microwaves at a power density of 1 mW/cm2 for 2 h/day over a period of 120, 150, and 200 days. Rearrangement of DNA segments were observed in testis and brain of exposed animals. Their data also suggested breakage of DNA strands after RFR exposure. Phillips et al. [21] were the first to study the effects of two forms of cell cellular phone signals, known as TDMA and iDEN, on DNA damage in Molt-4 human lymphoblastoid cells using the comet assay. These cells were exposed to relatively low intensities of the fields (2.4–26 μW/g) for 2–21 h. They reported both increased and decreased DNA damage, depending on the type of signal studied, as well as the intensity and duration of exposure. They speculated that the fields may affect DNA repair in cells. Subsequently, different groups of researchers have also reported DNA damage in various types of cells after exposure to cell phone frequency fields. Diem et al. [22] exposed human fibroblasts and rat granulosa cells to cell phone signal (1800 MHz; SAR 1.2 or 2 W/kg; different modulations; for 4, 16 and 24 h; intermittent 5 min on/10 min off or continuous). RFR exposure induced DNA single- and double-strand breaks as measured by the comet assay. Effects occurred after 16 h of exposure to different cell phone modulations in both cell types. The intermittent exposure schedule caused a significantly stronger effect than continuous exposure. Gandhi and Anita [23] reported increases in DNA strand breaks and micronucleation in lymphocytes obtained from cell phone users. Markova et al. [24] reported that GSM signals affected chromatin conformation and γ-H2AX foci that co-localized in distinct foci with DNA double-strand breaks in human lymphocytes. The effect was found to be dependent on carrier frequency. Nikolova et al. [25] reported a low and transient increase in DNA double-strand breaks in mouse embryonic stem cells after acute exposure to a 1.7-GHz field. Lixia et al. [26] reported an increase in DNA damage in human lens epithelial cells at 0 and 30 min after 2 h of exposure to a 1.8-GHz field at 3 W/kg. Sun et al. [27] reported an increase in DNA single-strand breaks in human lens epithelial cells after 2 h of exposure to a 1.8-GHz field at SARs of 3 and 4 W/kg. DNA damage caused by the field at 4 W/kg was irreversible. Zhang et al. [28] reported that an 1800-MHz field at 3.0 W/kg induced DNA damage in Chinese hamster lung cells after 24 h of exposure. Aitken et al. [29] exposed mice to a 900-MHz RFR at a SAR of 0.09 W/kg for 7 days at 12 h per day. DNA damage in caudal epididymal spermatozoa was assessed by quantitative PCR (QPCR) as well as by alkaline and pulsed-field gel electrophoresis. Gel electrophoresis revealed no significant change in single- or double-strand breaks in spermatozoa. However, QPCR revealed statistically significant damage to both the mitochondrial genome and the nuclear β-globin locus. Changes in sperm cell genome after exposure to 2450-MHz microwaves have also been reported previously by Sarkar et al. [20]. Related to this are several publications that have reported decreased motility and changes in morphology in isolated sperm cells exposed to cell phone radiation [30], sperm cells from animals exposed to cell phone radiation [31], and cell phone users [32], [33] and [34]. Some of these in vivo effects could be caused by hormonal changes [35] and [36].
There also are studies reporting no significant effect of cell phone RFR exposure on DNA damage. After RFR-induced DNA damage was reported by Lai and Singh [16] using 2450-MHz microwaves and after the report of Phillips et al. [21] on cell phone radiation was published, Motorola funded a series of studies by Roti Roti and colleagues [37] at Washington University to investigate DNA strand breaks in cells and animals exposed to RFR. None of the studies reported by this group found significant effects of RFR exposure on DNA damage [38], [39] and [40]. However, a different version of the comet assay was used in these studies. More recently, four additional studies from the Roti-Roti laboratories also reported no significant effects on DNA damage in cells exposed to RFR. Li et al. [41] reported no significant change in DNA strand breaks in murine C3H10T1/2 fibroblasts after 2 h of exposure to 847.74- and 835.02-MHz fields at 3–5 W/kg. Hook et al. [42] showed that a 24-h exposure of Molt-4 cells to CDMA, FDMA, iDEN or TDMA-modulated RFR did not significantly alter the level of DNA damage. Lagroye et al. [43] and [44] also reported no significant change in DNA strand breaks, protein–DNA crosslinks, and DNA–DNA crosslinks in cells exposed to 2450-MHz RFR.
From other laboratories, Vijayalaxmi et al. [45] reported no increase in DNA stand breaks in human lymphocytes exposed in vitro to 2450-MHz RFR at 2.135 W/kg for 2 h. Tice et al. [46] measured DNA single-strand breaks in human leukocytes using the comet assay after exposure to various forms of cell phone signals. Cells were exposed for 3 or 24 h at average SARs of 1.0–10.0 W/kg. Exposure for either 3 or 24 h did not induce a significant increase in DNA damage in leukocytes. McNamee et al. [47], [48] and [49] found no significant increase in DNA breaks and micronucleus formation in human leukocytes exposed for 2 h to a 1.9-GHz field at SAR up to 10 W/kg. Zeni et al. [50] reported that a 2-h exposure to 900-MHz GSM signal at 0.3 and 1 W/kg did not significantly affect levels of DNA strand breaks in human leukocytes. Sakuma et al. [51] exposed human glioblastoma A172 cells and normal human IMR-90 fibroblasts from fetal lungs to cell phone radiation for 2 and 24 h. No significant changes in DNA strand breaks were observed up to a SAR of 800 mW/kg. Stronati et al. [52] showed that 24 h of exposure to 935-MHz GSM basic signal at 1 or 2 W/Kg did not cause DNA strand breaks in human blood cells. Verschaeve et al. [53] reported that long-term exposure (2 h/day, 5 days/week for 2 years) of rats to 900-MHz GSM signal at 0.3 and 0.9 W/kg did not significantly affect levels of DNA strand breaks in cells.
3. Extremely low frequency electromagnetic fields (ELF EMF) and DNA damage
To complete the picture, a few words on the effects of ELF EMF are required, since cell phones also emit these fields and they are another common form of non-ionizing EMF in our environment. Quite a number of studies have indicated that exposure to ELF EMF could lead to DNA damage [54], [55], [56], [57], [58], [59], [60], [61], [62], [63], [64], [65], [66], [67], [68] and [69]. In addition, two studies [70] and [71] have reported effects of ELF fields on DNA repair mechanisms. Free radicals and interaction with transitional metals (e.g., iron) [60], [62], [63] and [69] have also been implicated to play a role in the genotoxic effects observed after exposure to these fields.
4. Some considerations on the effects of EMF on DNA
From this brief literature survey, no consistent pattern of RFR exposure inducing changes in or damage to DNA in cells and organisms emerges. However, one can conclude that under certain conditions of exposure, RFR is genotoxic. Data available are mainly applicable only to radiation exposure that would be typical during cell phone use. Other than the study of Phillips et al. [21], there is no indication that RFR at levels that one can experience in the vicinity of base stations and RF-transmission towers could cause DNA damage.
Differences in experimental outcomes are expected since many factors could influence the outcome of experiments in EMF research. Any effect of EMF has to depend on the energy absorbed by a biological organism and on how the energy is delivered in space and time. Frequency, intensity, exposure duration, and the number of exposure episodes can affect the response, and these factors can interact with each other to produce different effects. In addition, in order to understand the biological consequence of EMF exposure, one must know whether the effect is cumulative, whether compensatory responses result, and when homeostasis will break down. The contributions of these factors have been discussed in a talk given by one us (HL) in Vienna, Austria in 1998 [72].
Radiation from cell phone transmission has very complex patterns, and signals vary with the type of transmission. Moreover, the technology is constantly changing. Research results from one types of transmission pattern may not be applicable to other types. Thus, differences in outcomes of the research on genotoxic effects of RFR could be explained by the many different exposure conditions used in the studies. An example is the study of Phillips et al. [21], which demonstrated that different cell phone signals could cause different effects on DNA (i.e., an increase in strand breaks after exposure to one type of signal and a decrease with another). This is further complicated by the fact that some of the studies listed above used poor exposure procedures with very limited documentation of exposure parameters, e.g., using an actual cell phone to expose cells and animals, thus rendering the data from these experiments as questionable.
Another source of influence on experimental outcome is the cell or organism studied. Many different biological systems were used in the genotoxicity studies. Different cell types [73] and organisms [74] and [75] may not all respond similarly to EMF.
Comment about the comet assay also is required, since it was used in many of the EMF studies to determine DNA damage. Different versions of the assay have been developed. These versions have different detection sensitivities and can be used to measure different aspects of DNA strand breaks. A comparison of data from experiments using different versions of the assay could be misleading. Another concern is that most of the comet assay studies were carried out by experimenters who had no prior experience with this technique and mistakes were made. For example, in the study by Lagroye et al. [43] to investigate the effect of PK digestion on DNA migration after RFR exposure, PK was added to a lysing solution containing the detergent Triton X-100, which would inactivate the enzyme. Our experience indicates that the comet assay is a very sensitive and requires great care to perform. Thus, different detection sensitivities could result in different laboratories, even if the same procedures are followed. One way to solve this problem of experimental variation is for each research team to report the sensitivity of their comet assay, e.g., the threshold of detecting strand breaks in human lymphocytes exposed to X-rays. This information has generally not been provided for EMF-genotoxicity studies. Interestingly, when such information was provided, a large range of sensitivities have been reported. Malyapa et al. [40] reported a detection level of 0.6 cGy of gamma radiation in human lymphocytes, whereas McNamee et al. [76] reported 10–50 cGy of X-irradiation in lymphocytes, which is much higher than the generally acceptable detection level of the comet assay [15].
A drawback in the interpretation and understanding of experimental data from bioelectromagnetics research is that there is no general acceptable mechanism on how EMF affects biological systems. The mechanism by which EMF produces changes in DNA is unknown. Since the energy level associated with EMF exposure is not sufficient to cause direct breakage of chemical bonds within molecules, the effects are probably indirect and secondary to other induced biochemical changes in cells.
One possibility is that DNA is damaged by free radicals that are formed inside cells. Free radicals affect cells by damaging macromolecules, such as DNA, protein, and membrane lipids. Several reports have indicated that EMF enhances free radical activity in cells [18], [19], [61], [62], [77] and [78], particularly via the Fenton reaction [62]. The Fenton reaction is a process catalyzed by iron in which hydrogen peroxide, a product of oxidative respiration in the mitochondria, is converted into hydroxyl free radicals, which are very potent and cytotoxic molecules (Fig. 1).
Full-size image (8K)
Fig. 1. A representation of the Fenton reaction and its role as a mediator in EMF-induced bioeffects.
View Within Article
It is interesting that ELF EMF has also been shown to cause DNA damage. Furthermore, free radicals have been implicated in this effect of ELF EMF. This further supports the view that EMF affects DNA via an indirect secondary process, since the energy content of ELF EMF is much lower than that of RFR. Effects via the Fenton reaction predict how a cell would respond to EMF. For instance:
(1) Cells that are metabolically active would be more susceptible to EMF, because more hydrogen peroxide is generated by mitochondria to fuel the reaction.
(2) Cells that have high level of intracellular free iron would be more vulnerable to EMF. Cancer cells and cells undergoing abnormal proliferation have higher concentrations of free iron because they uptake more iron and have less efficient iron storage regulation. Thus, these cells could be selectively damaged by EMF. Consequently, this suggests that EMF could potentially be used for the treatment of cancer and hyperplastic diseases. The effect could be further enhanced if one could shift anaerobic glycolysis of cancer cells to oxidative glycolysis. There is quite a large database of information on the effects of EMF (mostly in the ELF range) on cancer cells and tumors. The data tend to indicate that EMF could ret*d tumor growth and kill cancer cells. One consequence of this consideration is that epidemiological studies of cancer incidence in cell phone users may not show a risk at all or even a protection effect.
(3) Since the brain is exposed to rather high levels of EMF during cell phone use, the consequences of EMF-induced genetic damage in brain cells are of particular importance. Brain cells have high levels of iron. Special molecular pumps are present on nerve cell nuclear membranes to pump iron into the nucleus. Iron atoms have been found to intercalate within DNA molecules. In addition, nerve cells have a low capacity for DNA repair, and DNA breaks could easily accumulate. Another concern is the presence of superparamagnetic iron-particles (magnetites) in body tissues, particularly in the brain. These particles could enhance free radical activity in cells and thus increase the cellular-damaging effects of EMF. These factors make nerve cells more vulnerable to EMF. Thus, the effect of EMF on DNA could conceivably be more significant on nerve cells than on other cell types of the body. Since nerve cells do not divide and are not likely to become cancerous, the more likely consequences of DNA damage in nerve cells include changes in cellular functions and in cell death, which could either lead to or accelerate the development of neurodegenerative diseases. Double-strand breaks, if not properly repaired, are known to lead to cell death. Cumulative DNA damage in nerve cells of the brain has been associated with neurodegenerative diseases, such as Alzheimer's, Huntington's, and Parkinson's diseases. However, another type of brain cell, the glial cell, can become cancerous as a result of DNA damage. The question is whether the damaged cells would develop into tumors before they are killed by EMF due to over accumulation of genetic damages. The outcome depends on the interplay of these different physical and biological factors—an increase, decrease, or no significant change in cancer risk could result from EMF exposure.
(4) On the other hand, cells with high amounts of antioxidants and antioxidative enzymes would be less susceptible to EMF. Furthermore, the effect of free radicals could depend on the nutritional status of an individual, e.g., availability of dietary antioxidants, consumption of alcohol, and amount of food consumption. Various life conditions, such as psychological stress and strenuous physical exercise, have been shown to increase oxidative stress and enhance the effect of free radicals in the body. Thus, one can also speculate that some individuals may be more susceptible to the effects of EMF exposure.
Additionally, the work of Blank and Soo [79] and Blank and Goodman [80] support the possibility that EMF exposure at low levels has a direct effect on electron transfer processes. Although the authors do not discuss their work in the context of EMF-induced DNA damage, the possibility exists that EMF exposure could produce oxidative damage to DNA.
5. Lessons learned
Whether or not EMF causes biological effects, let alone effects that are detrimental to human health and development, is a contentious issue. The literature in this area abounds with apparently contradictory studies, and as presented in this review, the literature specific to the effects of RFR exposure on DNA damage and repair in various biological systems is no exception. As a consequence of this controversy, there are several key issues that must be addressed—contrary data, weight of evidence, and data interpretation consistent with known science.
Consider that EMF does not share the familiar and comforting physical properties of chemical agents. EMF cannot be seen, tasted, smelled, or felt (except at high intensities). It is relevant, therefore, to ask, in what ways do scientists respond to data, especially if that data are contrary to their scientific beliefs or inconsistent with long-held hypotheses? Often such data are ignored, simply because it contradict what is accepted as conventional wisdom. Careful evaluation and interpretation of data may be difficult, because technologies used to expose biological systems to EMF and methodologies used to assess dosimetry generally are outside the experience of most biomedical scientists. Additionally, it is often difficult to assess differences in methodologies between studies, one or more of which were intended to replicate an original investigation. For instance, Malyapa et al. [40] reported what they claimed to be a replication of the work of Lai and Singh [16]. There were, however, significant differences in the comet analyses used by each group. Lai and Singh precipitated DNA in agarose so that low levels of DNA damage could be detected. Malyapa et al. did not. Lai and Singh treated their samples with PK to digest proteins bound to DNA, thus allowing DNA to move toward the positive pole during electrophoresis (unlike DNA, most proteins are negatively charged, and if they are not removed they will drag the DNA toward the negative pole). The Malyapa et al. study did not use PK. There were other methodological differences as well. Such is also the case in the study of Hook et al. [42], which attempted to replicate the work of Phillips et al. [21]. The latter group used a PK treatment in their comet assay, while the former group did not.
While credibility is enhanced when one can relate data to personal knowledge and scientific beliefs, it has not yet been determined how RFR couples with biological systems or by what mechanisms effects are produced. Even carefully designed and well executed RFR exposure studies may be summarily dismissed as methodologically unsound, or the data may be interpreted as invalid because of inconsistencies with what one believes to be correct. The quintessential example is the belief that exposure to RFR can produce no effects that are not related to the ability of RFR to produce heat, that is, to raise the temperature of biological systems [81] and [82]. Nonetheless, there are many examples of biological effects resulting from low-level (athermal) RFR exposure [83] and [84]. Consider here the work of Mashevich et al. [85]. This group exposed human peripheral blood lymphocytes to an 830-MHz signal for 72 h and at different average SARs (SAR, 1.6–8.8 W/kg). Temperatures ranged from 34.5 to 38.5 °C. This group observed an increase in chromosome 17 aneuploidy that varied linearly with SAR. Temperature elevation alone in the range of 34.5–38.5 °C did not produce this genotoxic effect, although significant aneuploidy was observed at higher temperatures of 40–41 °C. The authors conclude that the genotoxic effect of the radiofrequency signal used is elicited through a non-thermal pathway.
Also consider one aspect of the work of Phillips et al. [21]. In that study, DNA damage was found to vary in direction; that is, under some conditions of signal characteristics, signal intensity, and time of exposure, DNA damage increased as compared with concurrent unexposed controls, while under other conditions DNA damage decreased as compared with controls. The dual nature of Phillips et al.’s [21] results will be discussed later. For now consider the relationship of these results to other investigations. Adey et al. [86] performed an in vivo study to determine if rats treated in utero with the carcinogen ethylnitrosourea (ENU) and exposed to an 836.55-MHz field with North American Digital Cellular modulation (referred to as a TDMA field) would develop increased numbers of central system tumors. This group reported that rather than seeing an increase in tumor incidence in RFR-exposed rats, there was instead a decrease in tumor incidence. Moreover, rats that received no ENU but which were exposed to the TDMA signal also showed a decrease in the number of spontaneous tumors as compared with animals exposed to neither ENU nor the TDMA signal. This group postulated that their results may be mechanistically similar to the work of another group. Stammberger et al. [87] had previously reported that rats treated in utero with ENU and then exposed to low doses of X-irradiation exhibited significantly reduced incidences of brain tumors in adult life. Stammberger and colleagues [87] hypothesized that low-level X-irradiation produced DNA damage that then induced the repair enzyme 06-alkylguanine-DNA alkyltransferase (AT). Numerous groups have since reported that X-irradiation does indeed induce AT activity (e.g., [88] and [89]). In this context, it is significant that Phillips et al. [21] found that cells exposed in vitro to a TDMA signal identical to that used in the study of Adey et al. [86] produced a decrease in DNA damage under specific conditions of intensity and time of exposure (lower intensity, longer time; higher intensity, shorter time). These results raise the intriguing possibility that the decrease in tumor incidence in the study of Adey et al. [86] and the decrease in DNA damage in the study of Phillips et al. [21] both may have been the result of induction of AT activity resulting from DNA damage produced by exposure to the TDMA signal. This remains to be investigated.
Because the issue of RFR-induced bioeffects is contentious, and because the issue is tried in courtrooms and various public forums, a term heard frequently is weight of evidence. This term generally is used to describe a method by which all scientific evidence related to a causal hypothesis is considered and evaluated. This process is used extensively in matters of regulation, policy, and the law, and it provides a means of weighing results across different modalities of evidence. When considering the effects of RFR exposure on DNA damage and repair, modalities of evidence include studies of cells and tissues from laboratory animals exposed in vivo to RFR, studies of cells from humans exposed to RFR in vivo, and studies of cells exposed in vitro to RFR. While weight of evidence is gaining favor with regulators [90], its application by scientists to decide matters of science is often of questionable value. One of the reasons for this is that there generally is no discussion or characterization of what weight of evidence actually means in the context in which it is used. Additionally, the distinction between weight of evidence and strength of evidence often is lacking or not defined, and differences in methodologies between investigators are not considered. Consequently, weight of evidence generally amounts to what Krimsky [90] refers to as a “seat-of-the-pants qualitative assessment.” Krimsky points out that according to this view, weight of evidence is “a vague term that scientists use when they apply implicit, qualitative, and/or subjective criteria to evaluate a body of evidence.” Such is the case in the reviews by Juutilainen and Lang [91] and Verschaeve and Maes [92]. There is little emphasis on a critical analysis of similarities and differences in biological systems used, exposure regimens, data produced, and investigator's interpretations and conclusions. Rather, there is greater emphasis on the number of publications either finding or not finding an effect of RFR exposure on some endpoint. To some investigators, weight of evidence does indeed refer to the balance (or imbalance) between the number of studies producing apparently opposing results, without regard to critical experimental variables. While understanding the role these variables play in determining experimental outcome could provide remarkable insights into defining mechanisms by which RFR produced biological effects, few seem interested in or willing to delve deeply into the science.
A final lesson can be derived from a statement made by Gos et al. [93] referring to the work of Phillips et al. [21]. Gos and colleagues state, “The results in the latter study (Phillips et al., 1998) are puzzling and difficult to interpret, as no consistent increase or decrease in signal in the comet assay at various SARs or times of exposure was identified.” This statement is pointed out because studies of the biological effects of exposure to electromagnetic fields at any frequency are often viewed as outside of or distinct from what many refer to as mainstream science. However, what has been perceived as an inconsistent effect is indeed consistent with the observations of bimodal effects reported in hundreds of peer-reviewed publications. These bimodal effects may be dependent on concentration of an agent, time of incubation with an agent, or some other parameter relating to the state of the system under investigation. For instance, treatment of B cells for a short time (30 min) with the protein kinase C activator phorbol 12,13-dibutyrate increased proliferative responses to anti-immunoglobulin antibody, whereas treatment for a longer period of time (≥3 h) suppressed proliferation [94]. In a study of κ-opioid agonists on locomotor activity in mice, Kuzmin et al. [95] reported that higher, analgesic doses of κ-agonists reduced rearing, motility, and locomotion in non-habituated mice. In contrast, lower, subanalgesic doses increased motor activity in a time-dependent manner. Dierov et al. [96] observed a bimodal effect of all-trans-retinoic acid (RA) on cell cycle progression in lymphoid cells that was temporally related to the length of exposure to RA. A final example is found in the work of Rosenstein et al. [97]. This group found that the activity of melatonin on depolarization-induced calcium influx by hypothalamic synaptosomes from rats sacrificed late evening (2000 h) depended on melatonin preincubation time. A short preincubation time (10 min) stimulated uptake, while a longer preincubation (30 min) inhibited calcium uptake. These effects were also dependent on the time of day when the rats were sacrificed. Effects were maximal at 2000 h, minimal at 2400 h, and intermediate at 400 h. At 1000 h, only inhibitory effects of melatonin on calcium uptake were observed. These examples point out that what appears to be inconsistency may instead be real events related to and determined by the agents involved and the state of the biological system under investigation. The results of Phillips et al. [21] may be the result of signal modulation, signal intensity, time of exposure, or state of the cells. The results may indicate a bimodal effect, or they may, as the investigators suggest, represent time- and signal-dependant changes in the balance between damage and repair because of direct or indirect effects of RFR exposure on repair mechanisms.
6. Summary
Exposure of laboratory animals in vivo and of cultured cells in vitro to various radiofrequency signals has produced changes in DNA damage in some investigations and not in others. That many of the studies on both sides of this issue have been done well is encouraging from a scientific perspective. RFR exposure does indeed appear to affect DNA damage and repair, and the total body of available data contains clues as to conditions producing effects and methodologies to detect them. This view is in contrast to that of those who believe that studies unable to replicate the work of others are more credible than the original studies, that studies showing no effects cancel studies showing an effect, or that studies showing effects are not credible simply because we do not understand how those effects might occur. Some may be tempted to apply incorrectly the teachings of Sir Karl Popper, one of the great science philosophers of the 20th century. Popper proposed that many examples may lend support to an hypothesis, while only one negative instance is required to refute it [98]. While this holds most strongly for logical subjects, such as mathematics, it does not hold well for more complex biological phenomena that are influenced by stochastic factors. Each study to investigate RFR-induced DNA damage must be evaluated on its own merits, and then studies that both show effects and do not show effects must be carefully evaluated to define the relationship of experimental variables to experimental outcomes and to assess the value of experimental methodologies to detect and measure these outcomes (see Section 2).
The lack of a causal or proven mechanism(s) to explain RFR-induced effects on DNA damage and repair does not decrease the credibility of studies in the scientific literature that report effects of RFR exposure, because there are several plausible mechanisms of action that can account for the observed effects. The relationship between cigarette smoking and lung cancer was accepted long before a mechanism was established. This, however, occurred on the strength of epidemiologic data [99]. Fortunately, relevant epidemiologic data relating long-term cell phone use (>10 years) to central nervous system tumors are beginning to appear [84], [100], [101] and [102], and these data point to an increased risk of acoustic neuroma, glioma and parotid gland tumors.
One plausible mechanism for RFR-induced DNA damage is free radical damage. After finding that two free radical scavengers (melatonin and N-tert-butyl-α-phenylnitrone) prevent RFR-induced DNA damage in rat brain cells, Lai and Singh [62] hypothesized that this damage resulted from free radical generation. Subsequently, other reports appeared that also suggested free radical formation as a result of RFR exposure [103], [104] and [105]. Additionally, some investigators have reported that non-thermal exposure to RFR alters protein structure and function [106], [107], [108] and [109]. Scientists are familiar with molecules interacting with proteins through lock-and-key or induced-fit mechanisms. It is accepted that such interactions provide energy to change protein conformation and protein function. Indeed, discussions of these principles are presented in introductory biology and biochemistry courses. Perhaps then it is possible that RFR exposure, in a manner similar to that of chemical agents, provides sufficient energy to alter the structure of proteins involved in DNA repair mechanisms to the extent that their function also is changed. This has not yet been investigated.
When scientists maintain their beliefs in the face of contrary data, two diametrically opposed situations may result. On the one hand, data are seen as either right or wrong and there is no discussion to resolve disparities. On the other hand, and as Francis Crick [110] has pointed out, scientists who hold theoretically opposed positions may engage in fruitful debate to enhance understanding of underlying principles and advance science in general. While the latter certainly is preferable, there are external factors involving economics and politics that keep this from happening. It is time to acknowledge this and embark on the path of fruitful discussion. Great scientific discoveries await.
Acknowledgment
We thank Khushbu Komal and Ji-Sun Park for assistance in the preparation of the manuscript.
References
[1] J.F. Ward, The yield of DNA double strand breaks produced intracellularly by ionizing radiation: a review, Int. J. Radiat. Biol. 57 (1990), pp. 1141–1150. Full Text via CrossRef | View Record in Scopus | Cited By in Scopus (114)
[2] P.R. Walker, C. Smith, T. Youdale, J. Leblanc, J.F. Whitfield and M. Sikorska, Topoisomerase II-reactive chemotherapeutic drugs induce apoptosis in thymocytes, Cancer Res. 51 (1991), pp. 1078–1085. View Record in Scopus | Cited By in Scopus (236)
[3] Y. Onishi, Y. Azuma, Y. Sato, Y. Mizuno, T. Tadakuma and H. Kizaki, Topoisomerase inhibitors induce apoptosis in thymocytes, Biochem. Biophys. Acta 1175 (1993), pp. 147–154. Abstract | PDF (774 K) | View Record in Scopus | Cited By in Scopus (75)
[4] P. Prigent, C. Blanpied, J. Aten and F. Hirsch, A safe and rapid method for analyzing apoptosis-induced fragmentation of DNA extracted from tissues or cultured cells, J. Immunol. Methods 160 (1993), pp. 139–140. Abstract | PDF (113 K) | View Record in Scopus | Cited By in Scopus (54)
[5] K. Schindowski, S. Leutner, W.E. Muller and A. Eckert, Age related changes of apoptotic cell death in human lymphocytes, Neurobiol. Aging 21 (2000), pp. 661–670. Article | PDF (482 K) | View Record in Scopus | Cited By in Scopus (34)
[6] R.W. Hart and R.B. Setlow, Correlation between deoxyribonucleic acid excision repair and life span in a number of mammalian species, Proc. Natl. Acad. Sci. U.S.A. 71 (1974), pp. 2169–2173. Full Text via CrossRef | View Record in Scopus | Cited By in Scopus (154)
[7] M.R. Lieber, Pathological and physiological double-strand breaks: roles in cancer, aging, and theimmune system, Am. J. Pathol. 153 (1998), pp. 1323–1332. View Record in Scopus | Cited By in Scopus (72)
[8] H. Li, J.R. Mitchell and P. Hasty, DNA double-strand breaks: a potential causative factor for mammalian aging, Mech. Aging Dev. 129 (2008), pp. 416–424. Article | PDF (402 K) | View Record in Scopus | Cited By in Scopus (2)
[9] R.R. R.R.Tice, Aging and DNA repair capability. In: E.L. Schneider, Editor, The Genetics of Aging, Plenum Press, New York (1978), pp. 53–89.
[10] P.A. Cerutti, Peroxidant states and tumor promotion, Science 227 (1985), pp. 375–381. View Record in Scopus | Cited By in Scopus (979)
[11] B.N. Ames, Endogenous DNA damage as related to cancer and aging, Mutat. Res. 214 (1989), pp. 41–46. Abstract | PDF (458 K) | View Record in Scopus | Cited By in Scopus (134)
[12] B.N. Ames, Endogenous oxidative DNA damage, aging, and cancer, Free Radical Res. Commun. 7 (1989), pp. 121–128. Full Text via CrossRef | View Record in Scopus | Cited By in Scopus (331)
[13] T. Helleday, J. Loc, D.C. van Gentd and B.P. Engelward, DNA double-strand break repair: from mechanistic understanding to cancer treatment, DNA Repair 6 (2007), pp. 923–935. Article | PDF (840 K) | View Record in Scopus | Cited By in Scopus (61)
[14] R.B. Jarvis and J.F. Knowles, DNA damage in Zebrafish larvae induced by exposure to low-dose rate gamma-radiation: detection by the alkaline comet assay, Mutat. Res. 541 (2003), pp. 63–69. Article | PDF (161 K) | View Record in Scopus | Cited By in Scopus (8)
[15] R.R. Tice and G.H. Strauss, The single cell gel electrophoresis/comet assay: a potential tool for detecting radiation-induced DNA damage in humans, Stem Cells. Suppl. 1 (1995), pp. 207–214. View Record in Scopus | Cited By in Scopus (65)
[16] H. Lai and N.P. Singh, Acute low-intensity microwave exposure increases DNA single-strand breaks in rat brain cells, Bioelectromagnetics 16 (1995), pp. 207–210. Full Text via CrossRef | View Record in Scopus | Cited By in Scopus (172)
[17] H. Lai and N.P. Singh, Single and double-strand DNA breaks in rat brain cells after acute exposure to radiofrequency electromagnetic radiation, Int. J. Radiat. Biol. 69 (1996), pp. 513–521. Full Text via CrossRef | View Record in Scopus | Cited By in Scopus (187)
[18] H. Lai and N.P. Singh, Melatonin and a spin-trap compound block radiofrequency electromagnetic radiation-induced DNA strand breaks in rat brain cells, Bioelectromagnetics 18 (1997), pp. 446–454. Full Text via CrossRef | View Record in Scopus | Cited By in Scopus (77)
[19] H. Lai and N.P. Singh, Effects of microwaves and a temporally incoherent magnetic field on single and double DNA strand breaks in rat brain cells, Electromag. Biol. Med. 24 (2005), pp. 23–29. Full Text via CrossRef | View Record in Scopus | Cited By in Scopus (8)
[20] S. Sarkar, S. Ali and J. Behari, Effect of low power microwave on the mouse genome: a direct DNA analysis, Mutat. Res. 320 (1994), pp. 141–147. Abstract | PDF (538 K) | View Record in Scopus | Cited By in Scopus (70)
[21] J.L. Phillips, O. Ivaschuk, T. Ishida-Jones, R.A. Jones, M. Campbell-Beachler and W. Haggren, DNA damage in Molt-4 T- lymphoblastoid cells exposed to cellular telephone radiofrequency fields in vitro, Bioelectrochem. Bioenerg. 45 (1998), pp. 103–110. Article | PDF (100 K) | View Record in Scopus | Cited By in Scopus (53)
[22] E. Diem, C. Schwarz, F. Adlkofer, O. Jahn and H. Rudiger, Non-thermal DNA breakage by mobile-phone radiation (1800-MHz) in human fibroblasts and in transformed GFSH-R17 rat granulosa cells in vitro, Mutat. Res. 583 (2005), pp. 178–183. Article | PDF (268 K) | View Record in Scopus | Cited By in Scopus (57)
[23] G. Gandhi and Anita, Genetic damage in mobile phone users: some preliminary findings, Indian J. Hum. Genet. 11 (2005), pp. 99–104. View Record in Scopus | Cited By in Scopus (9)
[24] E. Markova, L. Hillert, L. Malmgren, B.R. Persson and I.Y. Belyaev, Microwaves from GSM mobile telephones affect 53BP1 and gamma-H2AX foci in human lymphocytes from hypersensitive and healthy persons, Environ. Health Perspect. 113 (2005), pp. 1172–1177. View Record in Scopus | Cited By in Scopus (17)
[25] T. Nikolova, J. Czyz, A. Rolletschek, P. Blyszczuk, J. Fuchs, G. Jovtchev, J. Schuderer, N. Kuster and A.M. Wobus, Electromagnetic fields affect transcript levels of apoptosis-related genes in embryonic stem cell-derived neural progenitor cells, FASEB J. 19 (2005), pp. 1686–1688. View Record in Scopus | Cited By in Scopus (31)
[26] S. Lixia, K. Yao, W. Kaijun, L. Deqiang, H. Huajun, G. Xiangwei, W. Baohong, Z. Wei, L. Jianling and W. Wei, Effects of 1.8-GHz radiofrequency field on DNA damage and expression of heat shock protein 70 in human lens epithelial cells, Mutat. Res. 602 (2006), pp. 135–142. Article | PDF (324 K) | View Record in Scopus | Cited By in Scopus (20)
[27] L.X. Sun, K. Yao, J.L. He, D.Q. Lu, K.J. Wang and H.W. Li, Effect of acute exposure to microwave from mobile phone on DNA damage and repair of cultured human lens epithelial cells in vitro, Zhonghua Lao Dong Wei Sheng Zhi Ye Bing Za Zhi 24 (2006), pp. 465–467. View Record in Scopus | Cited By in Scopus (1)
[28] D.Y. Zhang, Z.P. Xu, H. Chiang, D.Q. Lu and Q.L. Zeng, Effects of GSM 1800 MHz radiofrequency electromagnetic fields on DNA damage in Chinese hamster lung cells, Zhonghua Yu Fang Yi Xue Za Zhi 40 (2006), pp. 149–152. View Record in Scopus | Cited By in Scopus (6)
[29] R.J. Aitken, L.E. Bennetts, D. Sawyer, A.M. Wiklendt and B.V. King, Impact of radio frequency electromagnetic radiation on DNA integrity in the male germline, Int. J. Androl. 28 (2005), pp. 171–179. Full Text via CrossRef | View Record in Scopus | Cited By in Scopus (34)
[30] O. Erogul, E. Oztas, I. Yildirim, T. Kir, E. Aydur, G. Komesli, H.C. Irkilata, M.K. Irmak and A.F. Peker, Effects of electromagnetic radiation from a cellular phone on human sperm motility: an in vitro study, Arch. Med. Res. 37 (2006), pp. 840–843. Article | PDF (87 K) | View Record in Scopus | Cited By in Scopus (12)
[31] J.G. Yan, M. Agresti, T. Bruce, Y.H. Yan, A. Granlund and H.S. Matloub, Effects of cellular phone emissions on sperm motility in rats, Fertil. Steril. 88 (2007), pp. 957–964. Article | PDF (706 K) | View Record in Scopus | Cited By in Scopus (10)
[32] A. Agarwal, F. Deepinder, R.K. Sharma, G. Ranga and J. Li, Effect of cell phone usage on semen analysis in men attending infertility clinic: an observational study, Fertil. Steril. 89 (2008), pp. 124–128. Article | PDF (181 K) | View Record in Scopus | Cited By in Scopus (10)
[33] I. Fejes, Z. Za Vaczki, J. Szollosi, R.S. Kolosza, J. Daru, C.L. Kova and L.A. Pa, Is there a relationship between cell phone use and semen quality?, Arch. Androl. 51 (2005), pp. 385–393. Full Text via CrossRef | View Record in Scopus | Cited By in Scopus (27)
[34] A. Wdowiak, L. Wdowiak and H. Wiktor, Evaluation of the effect of using mobile phones on male fertility, Ann. Agric. Environ. Med. 14 (2007), pp. 169–172. View Record in Scopus | Cited By in Scopus (8)
[35] Z. Forgacs, Z. Somosy, G. Kubinyi, J. Bakos, A. Hudak, A. Surjan and G. Thuroczy, Effect of whole-body 1800 MHz GSM-like microwave exposure on testicular steroidogenesis and histology in mice, Reprod. Toxicol. 22 (2006), pp. 111–117. Article | PDF (235 K) | View Record in Scopus | Cited By in Scopus (5)
[36] M. Ozguner, A. Koyu, G. Cesur, M. Ural, F. Ozguner, A. Gokcimen and N. Delibas, Biological and morphological effects on the reproductive organ of rats after exposure to electromagnetic field, Saudi Med. J. 26 (2005), pp. 405–410. View Record in Scopus | Cited By in Scopus (18)
[37] J.L. Roti Roti, R.S. Malyapa, K.S. Bisht, E.W. Ahern, E.G. Moros, W.F. Pickard and W.L. Straube, Neoplastic transformation in C3H 10T(1/2) cells after exposure to 835.62 MHz FDMA and 847.74 MHz CDMA radiations, Radiat. Res. 155 (2001), pp. 239–247. Full Text via CrossRef | View Record in Scopus | Cited By in Scopus (19)
[38] R.S. Malyapa, E.W. Ahern, W.L. Straube, E.G. Moros, W.F. Pickard and J.L. Roti Roti, Measurement of DNA damage after exposure to 2450 MHz electromagnetic radiation, Radiat. Res. 1481 (1997), pp. 608–617. Full Text via CrossRef | View Record in Scopus | Cited By in Scopus (63)
[39] R.S. Malyapa, E.W. Ahern, W.L. Straube, E.G. Moros, W.F. Pickard and J.L. Roti Roti, Measurement of DNA damage after exposure to electromagnetic radiation in the cellular phone communication frequency band (835.62 and 847.74 MHz), Radiat. Res. 148 (1997), pp. 618–627. Full Text via CrossRef | View Record in Scopus | Cited By in Scopus (79)
[40] R.S. Malyapa, C. Bi, E.W. Ahern and J.L. Roti Roti, Detection of DNA damage by the alkaline comet assay after exposure to low-dose gamma radiation, Radiat. Res. 149 (1998), pp. 396–400. Full Text via CrossRef | View Record in Scopus | Cited By in Scopus (46)
[41] L. Li, K.S. Bisht, I. LaGroye, P. Zhang, W.L. Straube, E.G. Moros and J.L. Roti Roti, Measurement of DNA damage in mammalian cells exposed in vitro to radiofrequency fields at sars of 3–5 W/kg, Radiat. Res. 156 (2001), pp. 328–332. Full Text via CrossRef | View Record in Scopus | Cited By in Scopus (32)
[42] G.J. Hook, P. Zhang, I. Lagroye, L. Li, R. Higashikubo, E.G. Moros, W.L. Straube, W.F. Pickard, J.D. Baty and J.L. Roti Roti, Measurement of DNA damage and apoptosis in molt-4 cells after in vitro exposure to radiofrequency radiation, Radiat. Res. 161 (2004), pp. 193–200. Full Text via CrossRef | View Record in Scopus | Cited By in Scopus (46)
[43] I. Lagroye, R. R.Anane, B.A. Wettring, E.G. E.G.Moros, W.L. Straube, M. Laregina, M. Niehoff, W.F. Pickard, J. Baty and J.L. Roti, Measurement of DNA damage after acute exposure to pulsed-wave 2450 MHz microwaves in rat brain cells by two alkaline comet assay methods, Int. J. Radiat. Biol. 80 (2004), pp. 11–20. View Record in Scopus | Cited By in Scopus (19)
[44] I. Lagroye, G.J. Hook, B.A. Wettring, J.D. Baty, E.G. Moros, W.L. Straube and J.L. Roti Roti, Measurements of alkali-labile DNA damage and protein-DNA crosslinks after 2450 MHz microwave and low-dose gamma irradiation in vitro, Radiat. Res. 161 (2004), pp. 201–214. Full Text via CrossRef | View Record in Scopus | Cited By in Scopus (14)
[45] Vijayalaxmi, B.Z. Leal, M. Szilagyi, T.J. Prihoda and M.L. Meltz, Primary DNA damage in human blood lymphocytes exposed in vitro to 2450 MHz radiofrequency radiation, Radiat. Res. 153 (2000), pp. 479–486. View Record in Scopus | Cited By in Scopus (29)
[46] R.R. Tice, G.G. Hook, M. Donner, D.I. McRee and A.W. Guy, Genotoxicity of radiofrequency signals. I. Investigation of DNA damage and micronuclei induction in cultured human blood cells, Bioelectromagnetics 23 (2002), pp. 113–126. Full Text via CrossRef | View Record in Scopus | Cited By in Scopus (84)
[47] J.P. McNamee, P.V. Bellier, G.B. Gajda, S.M. Miller, E.P. Lemay, B.F. Lavallee, L. Marro and A. Thansandote, DNA damage and micronucleus induction in human leukocytes after acute in vitro exposure to a 1.9 GHz continuous-wave radiofrequency field, Radiat. Res. 158 (2002), pp. 523–533. Full Text via CrossRef | View Record in Scopus | Cited By in Scopus (38)
[48] J.P. McNamee, P.V. Bellier, G.B. Gajda, B.F. Lavallee, E.P. Lemay, L. Marro and A. Thansandote, DNA damage in human leukocytes after acute in vitro exposure to a 1.9 GHz pulse-modulated radiofrequency field, Radiat. Res. 158 (2002), pp. 534–537. Full Text via CrossRef | View Record in Scopus | Cited By in Scopus (31)
[49] J.P. McNamee, P.V. Bellier, G.B. Gajda, B.F. Lavallee, L. Marro, E. Lemay and A. Thansandote, No evidence for genotoxic effects from 24 h exposure of human leukocytes to 1.9 GHz radiofrequency fields, Radiat. Res. 159 (2003), pp. 693–697. Full Text via CrossRef | View Record in Scopus | Cited By in Scopus (26)
[50] O. Zeni, M. Romano, A. Perrotta, M.B. Lioi, R. Barbieri, G. d’Ambrosio, R. Massa and M.R. Scarfi, Evaluation of genotoxic effects in human peripheral blood leukocytes following an acute in vitro exposure to 900 MHz radiofrequency fields, Bioelectromagnetics 26 (2005), pp. 258–265. Full Text via CrossRef | View Record in Scopus | Cited By in Scopus (19)
[51] N. Sakuma, Y. Komatsubara, H. Takeda, H. Hirose, M. Sekijima, T. Nojima and J. Miyakoshi, DNA strand breaks are not induced in human cells exposed to 2.1425 GHz band CW and W-CDMA modulated radiofrequency fields allocated to mobile radio base stations, Bioelectromagnetics 27 (2006), pp. 51–57. Full Text via CrossRef | View Record in Scopus | Cited By in Scopus (12)
[52] L. Stronati, A. Testa, J. Moquet, A. Edwards, E. Cordelli, P. Villan, C. Marino, A.M. Fresegna, M. Appolloni and D. Lloyd, 935 MHz cellular phone radiation. An in vitro study of genotoxicity in human lymphocytes, Int. J. Radiat. Biol. 82 (2006), pp. 339–346. Full Text via CrossRef | View Record in Scopus | Cited By in Scopus (11)
[53] L. Verschaeve, P. Heikkinen, G. Verheyen, U. Van Gorp, F. Boonen, F. Vander Plaetse, A. Maes, T. Kumlin, J. Maki-Paakkanen, L. Puranen and J. Juutilainen, Investigation of co-genotoxic effects of radiofrequency electromagnetic fields in vivo, Radiat. Res. 165 (2006), pp. 598–607. Full Text via CrossRef | View Record in Scopus | Cited By in Scopus (11)
[54] Y.R. Ahuja, B. Vijayashree, R. Saran, E.L. Jayashri, J.K. Manoranjani and S.C. Bhargava, In vitro effects of low-level, low-frequency electromagnetic fields on DNA damage in human leucocytes by comet assay, Indian J. Biochem. Biophys. 36 (1999), pp. 318–322. View Record in Scopus | Cited By in Scopus (12)
[55] J. Delimaris, S. Tsilimigaki, N. Messini-Nicolaki, E. Ziros and S.M. Piperakis, Effects of pulsed electric fields on DNA of human lymphocytes, Cell Biol. Toxicol. 22 (2006), pp. 409–415. Full Text via CrossRef | View Record in Scopus | Cited By in Scopus (3)
[56] R. Hong, Y. Zhang, Y. Liu and E.Q. Weng, Effects of extremely low frequency electromagnetic fields on DNA of testicular cells and sperm chromatin structure in mice, Zhonghua Lao Dong Wei Sheng Zhi Ye Bing Za Zhi. 23 (2005), pp. 414–417. View Record in Scopus | Cited By in Scopus (3)
[57] S. Ivancsits, E. Diem, A. Pilger, H.W. Rudiger and O. Jahn, Induction of DNA strand breaks by intermittent exposure to extremely-low-frequency electromagnetic fields in human diploid fibroblasts, Mutat. Res. 519 (2002), pp. 1–13. Article | PDF (759 K) | View Record in Scopus | Cited By in Scopus (66)
[58] S. Ivancsits, E. Diem, O. Jahn and H.W. Rudiger, Age-related effects on induction of DNA strand breaks by intermittent exposure to electromagnetic fields, Mech. Aging Dev. 124 (2003), pp. 847–850. Article | PDF (300 K) | View Record in Scopus | Cited By in Scopus (17)
[59] S. Ivancsits, A. Pilger, E. Diem, O. Jahn and H.W. Rudiger, Cell type-specific genotoxic effects of intermittent extremely low-frequency electromagnetic fields, Mutat. Res. 583 (2005), pp. 184–188. Article | PDF (151 K) | View Record in Scopus | Cited By in Scopus (29)
[60] J. Jajte, M. Zmyslony, J. Palus, E. Dziubaltowska and E. Rajkowska, Protective effect of melatonin against in vitro iron ions and 7 mT 50 Hz magnetic field-induced DNA damage in rat lymphocytes, Mutat. Res. 483 (2001), pp. 57–64. Article | PDF (182 K) | View Record in Scopus | Cited By in Scopus (17)
[61] H. Lai, N.P. Singh and Melatonin, N-tert-butyl-alpha-phenylnitrone block 60-Hz magnetic field-induced DNA single and double strand breaks in rat brain cells, J. Pineal. Res. 22 (1997), pp. 152–162. Full Text via CrossRef | View Record in Scopus | Cited By in Scopus (72)
[62] H. Lai and N.P. Singh, Magnetic-field-induced DNA strand breaks in brain cells of the rat, Environ. Health Perspect. 112 (2004), pp. 687–694. View Record in Scopus | Cited By in Scopus (67)
[63] R. Lourencini da Silva, F. Albano, L.R. Lopes dos Santos, A.D. Tavares Jr. and I. Felzenszwalb, The effect of electromagnetic field exposure on the formation of DNA lesions, Redox. Rep. 5 (2000), pp. 299–301. Full Text via CrossRef | View Record in Scopus | Cited By in Scopus (7)
[64] C. Schmitz, E. Keller, T. Freuding, J. Silny and H. Korr, 50-Hz magnetic field exposure influences DNA repair and mitochondrial DNA synthesis of distinct cell types in brain and kidney of adult mice, Acta Neuropathol. (Berl) 107 (2004), pp. 257–264. Full Text via CrossRef | View Record in Scopus | Cited By in Scopus (3)
[65] B.M. Svedenstal, K.J. Johanson and K.H. Mild, DNA damage induced in brain cells of CBA mice exposed to magnetic fields, In Vivo 13 (1999), pp. 551–552. View Record in Scopus | Cited By in Scopus (22)
[66] R. Winker, S. Ivancsits, A. Pilger, F. Adlkofer and H.W. Rudiger, Chromosomal damage in human diploid fibroblasts by intermittent exposure to extremely low-frequency electromagnetic fields, Mutat. Res. 585 (2005), pp. 43–49. Article | PDF (110 K) | View Record in Scopus | Cited By in Scopus (21)
[67] F.I. Wolf, A. Torsello, B. Tedesco, S. Fasanella, A. Boninsegna, M. D’Ascenzo, C. Grassi, G.B. Azzena and A. Cittadini, 50-Hz extremely low frequency electromagnetic fields enhance cell proliferation and DNA damage: possible involvement of a redox mechanism, Biochim. Biophys. Acta 1743 (2005), pp. 120–129. Article | PDF (540 K) | View Record in Scopus | Cited By in Scopus (47)
[68] B. Yokus, D.U. Cakir, M.Z. Akdag, C. Sert and N. Mete, Oxidative DNA damage in rats exposed to extremely low frequency electro magnetic fields, Free Radic. Res. 39 (2005), pp. 317–323. Full Text via CrossRef | View Record in Scopus | Cited By in Scopus (33)
[69] M. Zmyslony, J. Palus, J. Jajte, E. Dziubaltowska and E. Rajkowska, DNA damage in rat lymphocytes treated in vitro with iron cations and exposed to 7 mT magnetic fields (static or 50 Hz), Mutat. Res. 453 (2000), pp. 89–96. Article | PDF (291 K) | View Record in Scopus | Cited By in Scopus (37)
[70] K. Chow and W.L. Tung, Magnetic field exposure enhances DNA repair through the induction of DnaK/J synthesis, FEBS Lett. 478 (2000), pp. 133–136. Article | PDF (74 K) | View Record in Scopus | Cited By in Scopus (25)
[71] J.G. Robison, A.R. Pendleton, K.O. Monson, B.K. Murray and K.L. O’Neill, Decreased DNA repair rates and protection from heat induced apoptosis mediated by electromagnetic field exposure, Bioelectromagnetics 23 (2002), pp. 106–112. Full Text via CrossRef | View Record in Scopus | Cited By in Scopus (24)
[72] H. Lai, Neurological effects of radiofrequency electromagnetic radiation, Mobile Phones and Health, Symposium, October 25–28 University of Vienna, Austria (1998)
[73] A. Höytö, J. Juutilainen and J. Naarala, Ornithine decarboxylase activity is affected in primary astrocytes but not in secondary cell lines exposed to 872 MHz RF radiation, Int. J. Radiat. Biol. 83 (2007), pp. 367–374. Full Text via CrossRef | View Record in Scopus | Cited By in Scopus (7)
[74] L.E. Anderson, J.E. Morris, L.B. Sasser and W. Löscher, Effects of 50- or 60-hertz, 100 microT magnetic field exposure in the DMBA mammary cancer model in Sprague-Dawley rats: possible explanations for different results from two laboratories, Environ. Health Perspect. 108 (2000), pp. 797–802. View Record in Scopus | Cited By in Scopus (11)
[75] A.L. Di Carlo and T.A. Litovitz, Is genetics the unrecognized confounding factor in bioelectromagnetics? Flock-dependence of field-induced anoxia protection in chick embryos, Bioelectrochem. Bioenerg. 48 (1999), pp. 209–215. Article | PDF (113 K) | View Record in Scopus | Cited By in Scopus (15)
[76] J.P. McNamee, J.R. McLean, C.L. Ferrarotto and P.V. Bellier, Comet assay: rapid processing of multiple samples, Mutat. Res. 466 (2000), pp. 63–69. Article | PDF (147 K) | View Record in Scopus | Cited By in Scopus (57)
[77] B. Oral, M. Guney, F. Ozguner, N. Karahan, T. Mungan, S. Comlekci and G. Cesur, Endometrial apoptosis induced by a 900-MHz mobile phone: preventive effects of vitamins E and C, Adv. Ther. 23 (2006), pp. 957–973. Full Text via CrossRef | View Record in Scopus | Cited By in Scopus (5)
[78] M. Simkó, Cell type specific redox status is responsible for diverse electromagnetic field effects, Curr. Med. Chem. 14 (2007), pp. 1141–1152. Full Text via CrossRef | View Record in Scopus | Cited By in Scopus (5)
[79] M. Blank and L. Soo, Electromagnetic acceleration of the Belousov-Zhabotinski reaction, Bioelectrochemistry 61 (2003), pp. 93–97. Article | PDF (196 K) | View Record in Scopus | Cited By in Scopus (16)
[80] M. Blank and R. Goodman, A mechanism for stimulation of biosynthesis by electromagnetic fields: charge transfer in DNA and base pair separation, J. Cell. Physiol. 214 (2008), pp. 20–26. Full Text via CrossRef | View Record in Scopus | Cited By in Scopus (7)
[81] K.R. Foster and R. Glaser, Thermal mechanisms of interaction of radiofrequency energy with biological systems with relevance to exposure guidelines, Health Phys. 92 (2007), pp. 609–620. Full Text via CrossRef | View Record in Scopus | Cited By in Scopus (4)
[82] A. Peter, T. Valberg, E. van Deventer and M.H. Repacholi, Workgroup Report: Base Stations and Wireless Networks—Radiofrequency (RF) Exposures and Health Consequences, Environ. Health Perspect. 115 (2007), pp. 416–424.
[83] D. Krewski, B.W. Glickman, R.W. Habash, B. Habbick, W.G. Lotz, R. Mandeville, F.S. Prato, T. Salem and D.F. Weaver, Recent advances in research on radiofrequency fields and health: 2001–2003, J. Toxicol. Environ. Health, Part B 10 (2007), pp. 287–318. Full Text via CrossRef | View Record in Scopus | Cited By in Scopus (8)
[84] L. Hardell, M. Carlberg, F. Soderqvist, K.H. Mild and L.L. Morgan, Long-term use of cellular phones and brain tumors: increased risk associated with use for ≥10 years, Occup. Environ. Med. 64 (2007), pp. 626–632. Full Text via CrossRef | View Record in Scopus | Cited By in Scopus (28)
[85] M. Mashevich, D. Folkman, A. Kesar, A. Barbul, R. Korenstein, E. Jerby and L. Avivi, Exposure of human peripheral blood lymphocytes to electromagnetic fields associated with cellular phones leads to chromosomal instability, Bioelectromagnetics 24 (2003), pp. 82–90. Full Text via CrossRef | View Record in Scopus | Cited By in Scopus (61)
[86] W. Adey, C. Byus, C. Cain, R. Higgins and R. Jones, Spontaneous and nitrosourea-induced primary tumors of the central nervous system in Fischer 344 rats chronically exposed to 836 MHz modulated microwaves, Radiat. Res. 152 (1999), pp. 293–302. Full Text via CrossRef | View Record in Scopus | Cited By in Scopus (66)
[87] J. Stammberger, W. Schmahl and L. Nice, The effects of X-irradiation, N-ethyl-N-nitrosourea or combined treatment on 06-alkylguanine-DNA alkyltransferase activity in fetal rat brain and liver and the induction of CNS tumors, Carcinogenesis 11 (1990), pp. 219–222.
[88] J.A. Rafferty, A.R. Clarke, D. Sellappan, M.S. Koref, I.M. Frayling and G.P. Margison, Induction of murine O6-alkylguanine-DNA-alkyltransferase in response to ionising radiation is p53 gene dose dependent, Oncogene 12 (1996), pp. 693–697. View Record in Scopus | Cited By in Scopus (65)
[89] T. Grombacher, U. Eichhorn and B. Kaina, p53 is involved in regulation of the DNA repair gene O6-methylguanine-DNA methyltransferase (MGMT) by DNA damaging agents, Oncogene 17 (1998), pp. 845–851. View Record in Scopus | Cited By in Scopus (66)
[90] S. Krimsky, The weight of scientific evidence in policy and law, Am. J. Public Health 95 (2005), pp. S129–S136. Full Text via CrossRef | View Record in Scopus | Cited By in Scopus (14)
[91] J. Juutilainen and S. Lang, Genotoxic, carcinogenic, and teratogenic effects of electromagnetic fields, Introduction and overview, Mutat. Res 387 (1997), pp. 165–171. Article | PDF (114 K) | View Record in Scopus | Cited By in Scopus (27)
[92] L. Verschaeve and A. Maes, Genetic, carcinogenic and teratogenic effects of radiofrequency fields, Mutat. Res. 410 (1998), pp. 141–165. Article | PDF (190 K) | View Record in Scopus | Cited By in Scopus (83)
[93] P. Gos, B. Eicher, J. Kohli and W.D. Heyer, No mutagenic or recombinogenic effects of mobile phone fields at 900 MHz detected in the yeast saccharomyces cerevisiae, Bioelectromagnetics 21 (2000), pp. 515–523. Full Text via CrossRef | View Record in Scopus | Cited By in Scopus (17)
[94] J.J. Mond, N. Feuerstein, C.H. June, A.K. Balapure, R.I. Glazer, K. Witherspoon and M. Brunswick, Bimodal effect of phorbol ester on B cell activation, J. Biol. Chem. 266 (1991), pp. 4458–4463. View Record in Scopus | Cited By in Scopus (7)
[95] A. Kuzmin, J. Sandin, L. Terenius and S.O. Ogren, Dose- and time-dependent bimodal effects of k-opioid agonists on locomotor activity in mice, J. Pharmacol. Exp. Ther. 295 (2000), pp. 1031–1042. View Record in Scopus | Cited By in Scopus (29)
[96] J. Dierov, B.E. Sawaya, M. Prosniak and R.B. Gartenhaus, Retinoic acid modulates a bimodal effect on cell cycle progression in human adult T-cell leukemia cell, Clin. Cancer Res. 5 (1999), pp. 2540–2547. View Record in Scopus | Cited By in Scopus (11)
[97] R.E. Rosenstein, D.A. Golombek, B.I. Kanterewicz and D.P. Cardinali, Time-dependency for the bimodal effect of melatonin on calcium uptake in rat hypothalamus, J. Neurol. Trans. 85 (1991), pp. 1435–1463.
[98] K.R. Popper, Conjectures and Refutations: The Growth of Scientific Knowledge, Harper & Row, NY (1963).
[99] J. Siemiatycki, Synthesizing the lifetime history of smoking, Cancer Epidemiol. Biomarkers Prev. 14 (2005), pp. 2294–2295. Full Text via CrossRef | View Record in Scopus | Cited By in Scopus (3)
[100] M.J. Schoemaker, A.J. Swerdlow, A. Ahlbom, A. Auvinen, K.G. Blaasaas, E. Cardis, H.C. Christensen, M. Feychting, S.J. Hepworth, C. Johansen, L. Klaeboe, S. Lonn, P.A. McKinney, K. Muir, J. Raitanen, T. Salminen, J. Thomsen and T. Tynes, Mobile phone use and risk of acoustic neuroma: results of the Interphone case-control study in five North European countries, Brit. J. Cancer 93 (2005), pp. 842–848. Full Text via CrossRef | View Record in Scopus | Cited By in Scopus (65)
[101] S. Sadetzki, A. Certrit, A. Jarus-Hakak, E. Cardis, Y. Deutch and S. Duvdevani et al., Cellular phone use and risk of benign and malignant parotid gland tumors–a nationwide case-control study, Am. J. Epidemiol. 167 (4) (2008), pp. 457–467. Full Text via CrossRef | View Record in Scopus | Cited By in Scopus (12)
[102] A. Lahkola, A. Auvinen, J. Raitanen, M.J. Schoemaker, H.C. Christensen, M. Feychting, C. Johansen, L. Klaeboe, S. Lonn, A.J. Swerdlow, T. Tynes and T. Salminen, Mobile phone use and risk of glioma in 5 North European countries, Int. J. Cancer 120 (2007), pp. 1769–1775. Full Text via CrossRef | View Record in Scopus | Cited By in Scopus (43)
[103] F. Oktem, F. Ozguner, H. Mollaoglu, A. Koyu and E. Uz, Oxidative damage in the kidney induced by 900-MHz-emitted mobile phone: protection by melatonin, Arch. Med. Res. 36 (2005), pp. 350–355. Article | PDF (180 K) | View Record in Scopus | Cited By in Scopus (23)
[104] M. Yariktas, F. Doner, F. Ozguner, O. Gokalp, H. Dogru and N. Delibas, Nitric oxide level in the nasal and sinus mucosa after exposure to electromagnetic field, Otolaryngol. Head Neck Surg. 132 (2005), pp. 713–716. Article | PDF (99 K) | View Record in Scopus | Cited By in Scopus (7)
[105] F. Ozguner, Y. Bardak and S. Comlekci, Protectuve effects of melatonin and caffeic acid phenethyl ester against retinal oxidative stress in long-term use of mobile phone: a comparative study, Mol. Cell. Biochem. 282 (2006), pp. 83–88. Full Text via CrossRef | View Record in Scopus | Cited By in Scopus (16)
[106] M. Porcelli, G. Cacciapuoti, S. Fusco, R. Massa, G. d’Ambrosio, C. Bertoldo, M. De Rosa and V. Zappia, Non-thermal effects of microwaves on proteins: thermophilic enzymes as model system, FEBS Lett. 402 (1997), pp. 102–106. Article | PDF (647 K) | View Record in Scopus | Cited By in Scopus (64)
[107] H. Bohr and J. Bohr, Microwave enhanced kinetics observed in ORD studies of a protein, Bioelectromagnetics 21 (2000), pp. 68–72. View Record in Scopus | Cited By in Scopus (22)
[108] F. Mancinelli, M. Caraglia, A. Abbruzzese, G. d’Ambrosio, R. Massa and E. Bismuto, Non-thermal effects of electromagnetic fields at mobile phone frequency on the refolding of an intracellular protein: myoglobin, J. Cell. Biochem. 93 (2004), pp. 188–196. Full Text via CrossRef | View Record in Scopus | Cited By in Scopus (9)
[109] M. Caraglia, M. Marra, F. Mancinelli, G. d’Ambrosio, R. Massa, A. Giordano, A. Budillon, A. Abbruzzese and E. Bismuto, Electromagnetic fields at mobile phone frequency induce apoptosis and inactivation of the multi-chaperone complex in human epidermoid cancer cells, J. Cell. Biochem. 204 (2005), pp. 539–548. Full Text via CrossRef | View Record in Scopus | Cited By in Scopus (25)
[110] F. Crick, What Mad Pursuit: A Personal View of Scientific Discovery, Basic Books, New York (1988).
I am pretty sure that if this was true then we would see a higher than normal rate of brain cancer in teenagers and businessmen. And in Japan and Korea, where prolific cell phone use by everyone including preschoolers is probably 10 times what it is in the US.
and according to them, that is not the case
http://www.healthlibrary.com/news932.htm
and interestingly enough, malignant brain and CNS cancer in Japan has decreased over the last 20 years.
_________________
ADHD-diagnosed
Asperger's Syndrome-diagnosed
Similar Topics | |
---|---|
Phone |
18 Nov 2024, 10:18 am |
Phone thermal |
23 Oct 2024, 5:07 pm |
The Human Brain |
30 Nov 2024, 9:36 pm |
The Autistic Brain |
13 Dec 2024, 9:34 am |